4 different sources of DNA
To remake yourself in the image of who you want to be, you must understand who you are and the processes that shape you. Only understanding the mechanisms that shape you can you redesign yourself to be a better approximation of your ideal.
In this series ‘The Genetic Self’ I’ve been writing about the genetic information that governs protien synthesis in our bodies, and how it can program us for unconscious, automatic thoughts, feelings, and behaviors that are unlike those we would choose if we could.
Our genes act so fast that we often find ourselves reacting before we’ve had a chance to think things through. From the standpoint of evolution and reproductive fitness, that can be a big advantage. For example, when we’re confronted with danger, the sympathetic division of the autonomic nervous system activates the fight, flight, freeze, or fawn response. For example, our heart rate increases, epinephrine rushes through out our bodies, and our liver releases gylcogen into our bloodstream to prepare ourselves for combat.
That’s not a reactions we can typically control with our conscious mind, although their are breathing exercises that can regain control of the sympathetic activation, strengthen the parasympathetic (sometimes called rest & digest) and calm ourselves down.
Nonetheless, redesigning our genetic responses to be more in tune with the challenges we face today, rather than those our ancient ancestors evolved with, could be a tremendous boost to our success. Moreover, if we were able to delay the inevitable damage or decay that happens to genetic information as we age, we might find that our brains and bodies enjoy more high health quality years before we must surrender to ravages of time.
To understand our genetic selves, and how to reprogram the expression of our genes, we must first understand which genes govern out automatic systems and how. There are at least four different sources of genetic control that operate within your body:
Your nucleic DNA, inherited from your parents in their sperm and egg,
Your epigentic adaptations, which can be both inherited and acquired from experience,
Your microbiome, which are the bacteria and other microorganisms that live on our inside your body and make up about 99% of the genetic information on which you depend, and
Your mitochondria.
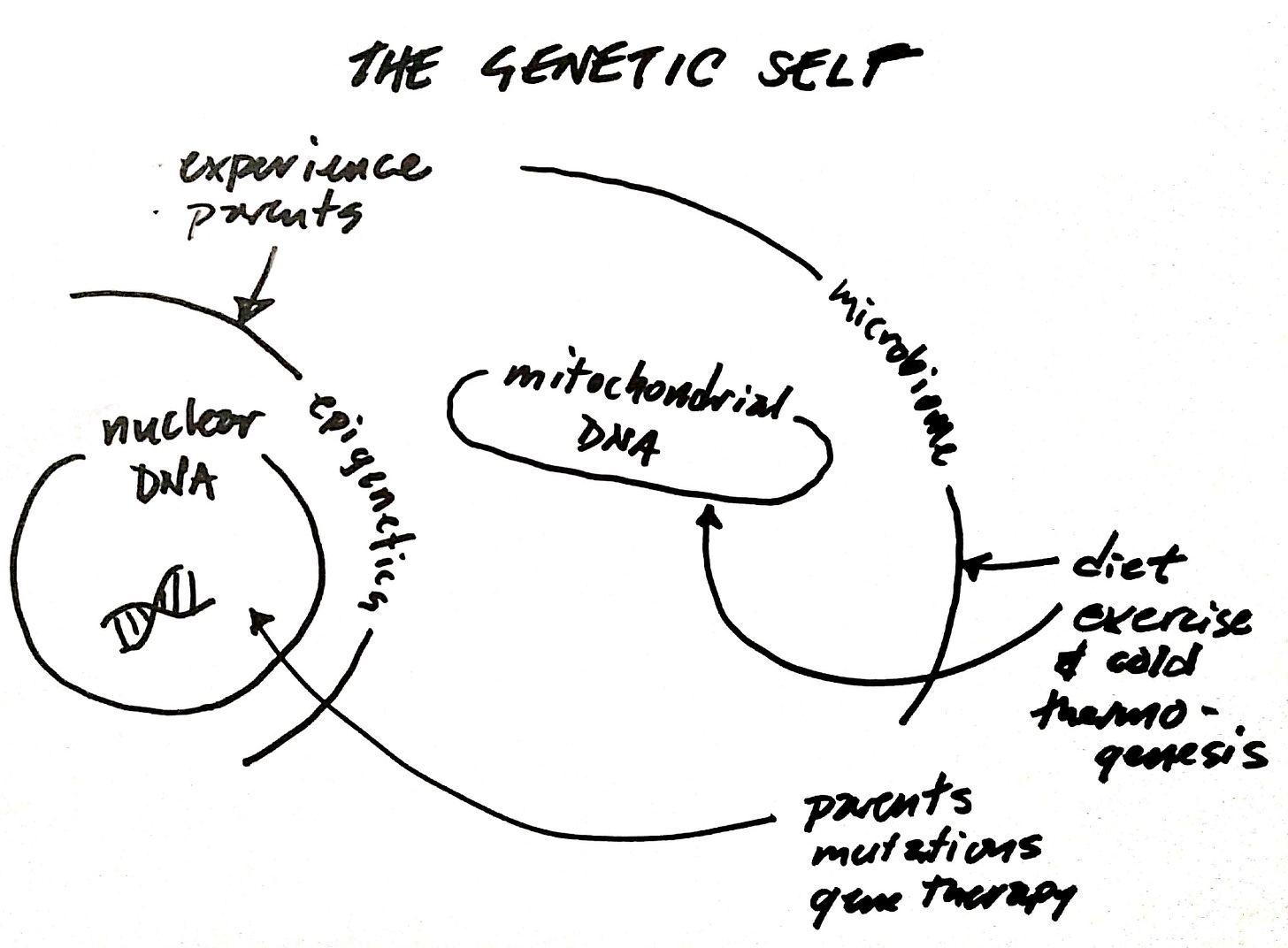
Nucleic DNA get all the attention. That’s what we study in school, and it does control the color of our eyes, or hair, and the visible features that make us look similar to our close relatives and create feelings of kinship. Nonetheless, in Debunking DNA I wrote about the dangerous myth that nucleic DNA is somehow the master controller of our destiny.
It’s not.
For example, the Human Genome Project has been a bust. Despite the hyperbolic expectations that accompanied its launch during the Clinton administration, mapping the human genome has not led to the revolution in personalized medicine that was promised. Although it has improved our understanding of genetics, it the principal outcome of the Human Genome Project has been to reinforce the realization that genetic expression is more complex than the Industrial Age understanding of DNA was giving it credit for.
For example, epigenetics are the mechanisms that switch on or off the expression of DNA. So epigenetic mechanisms, like coating DNA with methyl groups that prevent the action of underlying genes, can change the functional workings of the DNA if not the chemical structure of the double helix itself.
What’s even more amazing is that epigentics are controled by experience. Unresolved trauma, for example, can alter epigenetic expression of the underlying genes to make us better adapted to environmental or experiential stressors — and these epigenetic expressions can be passed down to our children, which is another way of saying that we can inherit trauma from our ancestors.
Of the 4 different types of DNA, the microbiome is the most flexible, most difficult to measure, and probably the easiest in which to intervene. However, this post is not about the microbiome. I’ll return to that topic later.
This post is about the DNA that controls your ageing: mitochondrial DNA.
Mitochondrial DNA
Energy = negative entropy
Inside every cell of our body, with the exception of red blood cells, there are hundreds of microscopic organelles called mitochondria. They are the site of energy conversion, which is to say that triglycerides (fats) and glucose travel from the blood stream, through the cell wall, to the mitochondria, where they are converted to ATP that fuels exercise, growth, and all the energetic processes of the body.
There can be no human life without functioning mitochondria, because life is energy.
While most theories of ageing focus on the damage and mutations that take place over time inside nucleic DNA (e.g., cancer), the mitochondrial DNA — being outside the nucleus — are ten times more vulnerable to harm and more difficult to repair.
Because mitochondria are essential to energy conversion, proper functioning of mitochondria are essential to life.
Life does not exist without energy. As the 2nd law of thermodynamics dictates, a constant throughput of energy is required to keep matter organized. Without energy, any system will inevitably degrade into a higher state of entropy — which is to say that matter will lose all structure and organization without energy to maintain it.
The only thing that keeps the body from its inevitable decay is the ATP produced by our mitochondria. When that stops, we’re dead.
While it is true that cancer is fatal to the host, it’s also interesting to point out that cancer cells are alive. In fact, it is their ravenous appetite for glucose that fuels their uncontrolled growth.
In other words, cancer is a metabolic disorder of the mitochondria first, and a disorder of nucleic DNA second.
Why should mitochondria have their own DNA?
The best explanation that I’ve seen so far says that energy production is so vital to the function of our bodies — especially in emergencies — that we can ill afford to have the enzymes essential for it synthesized in the nucleus and then wait for them to find their way to the mitochondria. That is, the mitochondria need their own DNA to ensure that the proteins they encode will be available when they are needed, where they are needed, without delay.
That makes sense to me… but I have my own, additional theory.
The problem with the proximal theory of mitochondira is that all the DNA and functions of the mitochondria could be incorporated into nucleic DNA, instead of kept outside. Why should energy production be outside the nucleus at all, instead of bringing it inside where the rest of our inherited DNA is found?
There could be several reasons for it that have been overlooked. The first is that energy production requires hundreds — sometimes even thousands — of copies of mitochondria per cell.
But there is only one nucleus.
Scaling up the nucleic DNA to handle the throughout demands currently met by hundreds of mitochondria working in parallel might never work. And even if it did, the scale of energy conversion operations, should they be located in the nucleus, might so enormous that they interfere with other nucleic functions.
Having mitochondria outside the nucleus allows greater flexibility in their function and scale. In cells like brown fat, that require a great deal of energy to maintain warm body temperatures in the cold, thousands of mitochondria are required. However, skeletal cells require fewer. Having mitochondria work indepedently of nucleic DNA affords each cell type greater flexibility and responsiveness for meeting diverse energy demands in different cell types.
And there is another reason to keep the mitichondria away from the nucleus, and that is because energy conversion is dangerous.
As electrons get passed around from triglycerides and glucose into ATP and the waste products of metabolism (carbon dioxide and water), it is inevitable that some of them get loose — especially when mitochondria are overheated or overloaded. These stray electrons from reactive oxygen species (ROS) that can damage DNA.
Having ROS in the cell nucleus could prove fatal. Keeping the mitochondria outside the nucleus allows for mitochondria to be damaged, detected, and replaced, without putting the nucleic DNA at risk.
Mitochondrial biogenesis
Mitochondrial DNA are inherited exclusively from Mothers. When a Father’s mitochondrial DNA gets involved, it leads to functional disorders that usually result in the death of the organism.
To ensure an exclusively maternal lineage, the mitochondrial DNA supplied to the zygote by the sperm are destroyed within hours of fertilization (Zhou et al. 2011).
It isn’t obvious why mitochondria inheritance should work this way. Metaphorically, mitochondria are like bees and ants — they’re all identical twin sisters.
And it turns out that mitochondria work together like a beehive, too. Sometimes mitochondria fuse together to form long, energetic engines, and sometimes they operate individually.
When the body needs new mitochondria, they aren’t synthesized by the DNA in the nucleus, or from stem cells created elsewhere in the body. They are formed from the genetic information resident in existing mitochondria in a process called mitobiogenesis.
Thus, the body has mechanisms for deleting old or defective mitochondria (called mitophagy) and replacing them with new.
Perhaps there is something about the exclusively matriarchal lineage of mitochondria that facilitates their coordination and reproduction, independent of the sexual reproduction that governs the union of egg and sperm. The implication is that we all carry mitochondrial DNA that is identical to Eve, the original human Mother — but that isn’t the case. Although mitochondrial DNA are more stable across generations, there are still mutations, and occasional insertions of paternal mitochondria DNA, that allow mitochondria DNA to evolve.
Ageing
300 different theories
Although science can answer questions related to how we age, and what happens when we age, it cannot discern why we should age. All we know is that ageing is inevitable, and that it is charaterized by predictable, physiological changes in the body.
Some of these changes might be a consequence of ageing, and some of them might be a cause of ageing. Distinguishing between the two is important for prolonging quality of life — i.e., lengthening the healthspan.
For example, iron tends to accumulate in the body over time — particularly in men. This is because we are constantly taking iron into our bodies in the foods we eat, but the only mechanism by which we lose iron is blood loss. Therefore, as we get older we tend to accumulate greater iron stores.
Is iron accumulation a consequence of ageing, resulting from the gradual accumulation of iron in the body over time? Or is iorn accumulation the cause of other changes in the body that are associated with ageing?
Increasingly, evidence suggests that iron accumulation accellerates ageing. That is, reducing iron levels via phlebotomy (blood donation) extends healthspan, which supports an iron accumulation theory of ageing.
According to one comprehensive review, there are over 300 theories of ageing (Medvedev 1990), including iorn accumulation and theories that focus on nucleic DNA (e.g., methylation and telomere length).
However, there is only one thing that has been proven in laboratory studies to reliably extend lifespan: caloric restriction.
Ageing is an energetic decline
The only theory that has produced reliable experimental anti-ageing success is energetic.
According to the energetic theory, ageing is caused by an accumulation of irreparable damage in mitochondrial DNA. When the mitochondria are no longer able to function, they stop converting energy, and the organism dies.
Caloric restriction prevents mitochondrial damage by reducing the mitochondrial load and slowing production of reactive oxygen species (ROS). That is, when we overeat, we stress the mitochondria by giving them too much food to process. That creates ROS that damage mitochondrial DNA, requiring mitophagy and mitobiogenesis.
According to this theory, without a period of fasting to allow the mitochondrial recovery processes, chronic energetic stress wears down the quality of our mitochondrial DNA until eventually they can no longer sustain life.
However, it’s not just any food that will overload our mitochondria. Refined carbohydrates — i.e., sugars — are the worst.
Eating sugar pushes up levels of glucose in the blood, and causes the islet cells in the pancreas to produce more insulin. For reasons that are opaque to me, glucose cannot move from the bloodstream into the cell membrane without the assistance of insulin. That’s why a Type 1 diabetic (like my son) who no longer produces insulin suffers from chronic hyperglycemia (high blood sugar) without regular injections of insulin.
When we eat more carbohydrates than our body demands for movement, growth, and thermogenesis (production of heat), that glucose must be converted to trigylcerides and stored in white fat cells.
Too many carbohydrates all at once will overwhelm and damage mitochondria. However, cells have a built-in mitochondrial defense mechanism called insulin resistance.
Occassional carbohydrate surges, followed by carb fasting to allow mitochondria recovery, are no big deal for the body. However, chronic overloading of carbohydrates, as is typical in the standard American diet (SAD) eventually causes the cells to slow transport of more glucose to the mitochondria by resisting the action of insulin. In that case, the glucose remains in the blood stream, giving the damaged mitochondria more time to process it.
When insulin resistance progresses to advanced stages, we call it Type 2 diabetes.
According to Ben Bikman, PhD insulin resistance is the underlying mechanism that results in 8 out of the 10 leading causes of death in the United States (Why We Get Sick, Bikman 2020). For example, insulin resistance is associated with Alzhiemer’s, cardio-vascular disease, fatty liver disease, and cancer.
If this energetic theory of ageing, also called the ROS theory, is true, then the way to extend healthspan is to:
limit intake of high carbohydrate, high gylcemic index foods.
periodically fast from carbohydrates, to allow mitochondrial recovery through mitophagy & mitobiogensis.
match carbohydrate intake to levels of exercise, growth (e.g., puberty), and thermogenesis.
promote mitochondrial health via nutrition, exercise, thermal contrast therapy (e.g., ice baths & sauna) and exercise.
As it turns out, every single one of these practices has been demonstrated to improve well-being and extend healthspan.
Incredible opening line: "To remake yourself in the image of who you want to be, you must understand who you are and the processes that shape you. Only understanding the mechanisms that shape you can you redesign yourself to be a better approximation of your ideal."
Reminds me of the Santiago Ramón y Cajal quote “Any man could, if he were so inclined, be the sculptor of his own brain.”