Summary
Genetics controls only 10% of ageing - the rest is lifestyle.
Experiences and exposures that damage mitochondria accelerate ageing.
A long lifespan requires protecting mitochondrial DNA from damage.
Cold therapy is one of the best things you can do for your mitochondria.
Add post-cold exercise & red light for an extra mitochondrial boost.
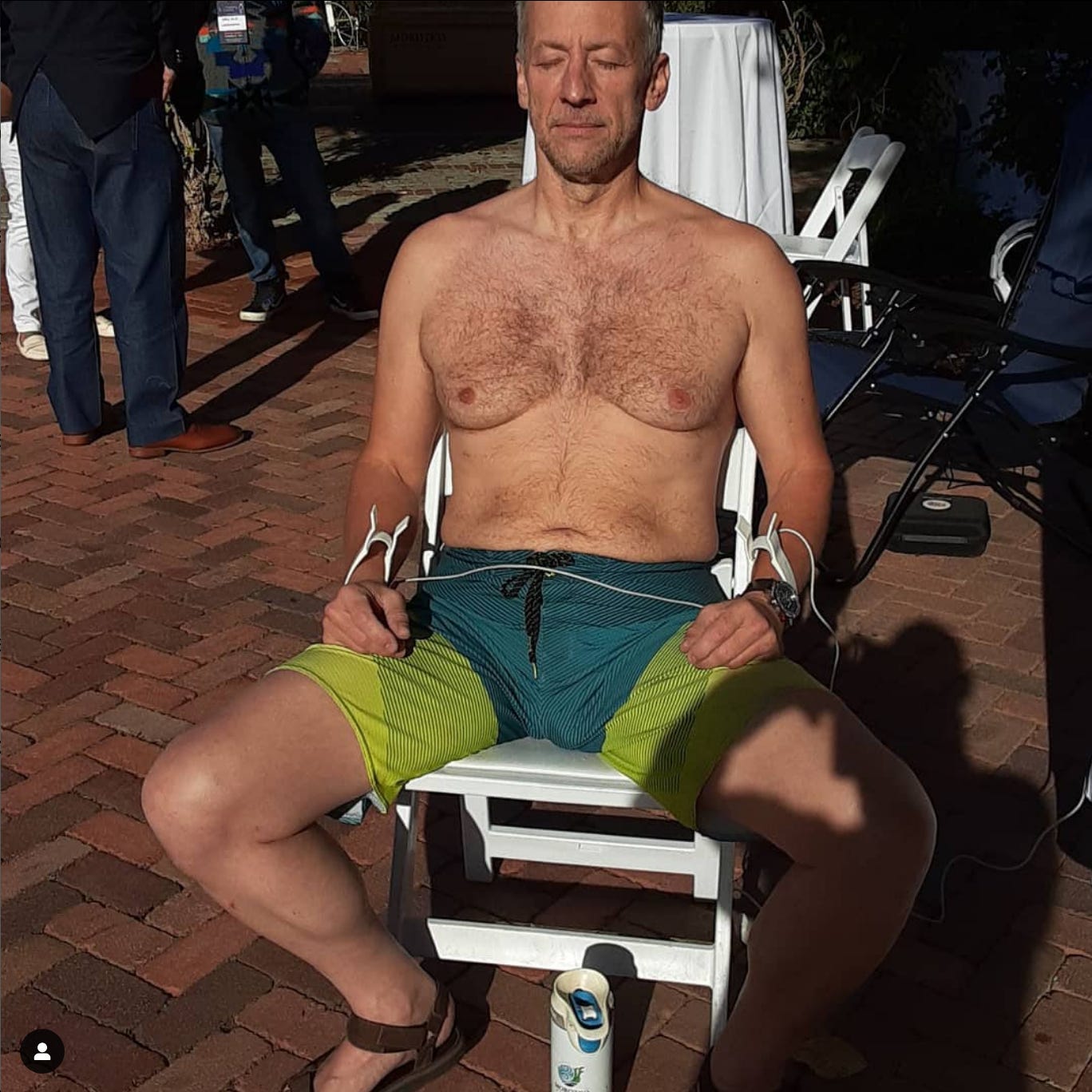
Chronological vs biological age
In The Genetic Self: Biological Age, I explained the difference between chronological age, which is measured by your birthday, and biological age, which is supposed to provide an improved estimate of your expected lifespan.
Here's an excerpt:
There are two criteria that any effective measure of biological age must demonstrate:
1. For large statistical ensembles, it must correlate with chronological age.
2. For individuals, the departures from chronological age correlation must be predictive of expected lifespan. Put another way, wherever biological age departs from chronological age, the biological must be a better predictor of mortality than chronological.
According to the Elysium Health ‘Index’ test, my biological age is 51 — five years younger than my chronological age. That feels good in the sense that it’s better than finding out that my DNA methylation is typical of someone older than me, but it doesn’t help me understand what I might do to take care better of myself.
A reliable measure of biological age would be an enormous boon to anyone seeking to slow the rate at which they age, extend their life- and healthspan, and manage their overall wellness.
I'm one of those people. At 56 years old, I've started thinking about how I might live a longer, healthier (and happier) life than my Father and Mother.
Dad was morbidly obese and riddled with cancer when he passed away peacefully in his sleep at the age of 91. Mom died younger and more recently, but she suffered from acute dementia that meant the quality of her last decade of life was poor.
My parents always told me that I could expect to live a long life, because I had "good genes." After all, didn't my Grandmother Harriett live until the age of 99?
It was only recently that I discovered how wrong that thinking was.
An extensive review of the data available in ancestry.com revealed that less than 10% of lifespan could be explained by genetics (Ruby et al. 2018). The remainder, say the researchers, is due to lifestyle choices and associated with socio-economic status -- which is also transferred within families from the older to younger generations.
For example, diet, exercise, smoking, and alcohol consumption modify expected lifespan. Neither my Father nor his Mother (my Nana) smoked our drank alcohol, and it's obvious that my Father had access to better healthcare, so why did Dad die seven years younger than she did (and a year younger than Papa)?
We don't know for sure, but maybe Nana's longevity was because she preferred steak. Like George H.W. Bush (1924 - 2018), who famously hated broccoli, nobody could make Nana eat her vegetables.
My Father, on the other hand, had a sweet tooth.
The problem with biomarkers of biological age
There are several chemical biomarker tests that you can order online that purport to assess your biological age. Like the DNA methylation test that I took, you can also measure your telomere length. Both of these tests examine chemical markers of genetic health and function. For example, telomeres are nucleotides that protect chromosomes during replication. The longer you live, the shorter your telomeres get, leaving your chromosomes more vulnerable to copying errors. In theory, shorter telomeres are indicative of older age.
The problem is that telomere length doesn't correlate with chronological age, and measuring it doesn't improve prediction of lifespan. In fact, none of the material biomarkers that might be hypothetically associated with ageing do a good job of estimating expected lifespan better than chronological age (Jansen et al. 2021).
So my Elysium Health estimate of 51 years doesn't mean a dang thing.
That's because all of the material biomarkers are examining the DNA in the cell nucleus, when the escapement that controls our biological clock is more likely found in the mitochondria.
As I explained in 4 Sources of DNA, the mitochondria contain their own DNA, independent of the DNA in your cell nucleus that you inherited from your parents. The mitochondria are called organelles, because they exist inside the cells of your body. Every cell in your body except red blood cells contains hundreds of mitochondria.
Brown fat cells contain thousands.
The mitochondria perform energy conversion for the body. They convert food substrates, glucose and fats, into electrical energy (carried via ATP) that can be used to move muscles, repair tissues, or fuel tissue growth and repair. So every function in the body depends on mitochondria for life.
But mitochondria are difficult to measure. They don't lend themselves to the same bioanalytic techniques that nucleic DNA do -- partly because there are so many of them. Each mitochondria contains multiple copies of mtDNA.
Why mitochondria?
Although mitochondria were first discovered in 1857, and their essential function in energy conversion was understood even then, their origins remained a mystery until the 1970's. That's when Lynn Margulis hypothesized that mitochondria were once independent, single-celled prokaryotic bacteria that got subsumed by more complex eukaryotes and, instead of being digested, were put to work to convert energy inside their more complex, eukaryotic host organisms.
The idea seems far-fetched, and Margulis' hypothesis was initially rejected and ridiculed, only later to become accepted as fact.
As prokaryotes, mitochondria are distinct from eukaryotes in two critical ways:
prokaryotes do not have a nucleus to protect their DNA, and that makes mtDNA more vulnerable to damage than the nucleic DNA inside eukaryotic cells, and
prokaryotes reproduce by cell division, such that two mitochondria with identical mtDNA can be formed from a single progenitor mitochondria. In eukaryotes, this process is called mitosis, and it supports growth within a single organism, but not reproduction, per se. Eukaryotes depend on sexual reproduction, whereas prokaryotes are asexual.
Human beings are eukaryotes, and that means that reproduction requires genes from both a Mother and a Father to create a new organism with unique DNA. When that creature dies, its DNA dies with it.
However, as prokaryotes, mitochondrial DNA is immortal. It can keep reproducing itself into identical copies a theoretically infinite number of times.
To avoid genetic confusion inside the mitochondria, only the Mother's mitochondrial DNA is preserved. Although sperm also carry their own mitochondrial DNA, these are normally destroyed after fertilization of the egg, so that the baby's mitochondria are identical to its Mother's, and Grandmother's, and great-great-great-Grandmother's -- going all the way back to Eve.
In practice, mutations and oddball paternal contributions do occur within a mitochondrial lineage, so mtDNA does evolve. However, when we say that mitochondria are immortal because of their prokaryotic origins, this is what we mean: your mtDNA will outlive you.
Keep reading with a 7-day free trial
Subscribe to Self-Actual Engineering to keep reading this post and get 7 days of free access to the full post archives.